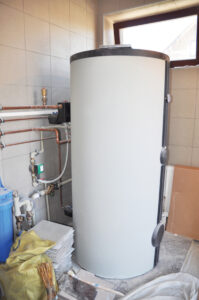
Hot Water Heating Boiler.
One of our Chemists, Raul Dacomba, wrote a white paper for Appliance Design.
Change is a certainty in this world, and the appliance industry is no exception. Regulatory mandates are imposing yet another transition to a new generation of polyurethane foam blowing agents. The legislation states that Hydrofluorocarbons (HFCs) and blends will be unacceptable for use starting January 2020. Refrigerant use will be unacceptable starting January 2021.
A blowing agent is one of many key ingredients used to manufacture polyurethane foams; their inherently low thermal conductivities are a desired trait. Before delving into specifics on the future of appliance foams, it is important to understand the history of blowing agents.
First Generation: CFCs (Chlorofluorocarbons)
In the days of Otto Bayer, water was the original blowing agent used for creating polyurethane foams; the reaction of water and isocyanate generates CO2, which serves as an expansion agent. Albeit the use of water to generate CO2 worked, it wasn’t until the inception of trichlorofluoromethane (CFC-11 or R-11), that polyurethane foams became a key player in insulation, especially in refrigeration. This liquid boiled at room temperature (75°F) and was non-toxic, non- corrosive, and non-flammable. R-11 brought several advantages to the formulators: excellent solubility with most polyols, viscosity reduction of isocyanates, and allowed for a more controllable and uniform reaction profile, to name a few. Most importantly, this foam blowing agent was more thermally efficient. R-11 had a gas lambda of 8.4 mW/m-K vs. 14.7 mW/m-K for CO2—the lower the gas lambda, the better insulation performance. This directly translated to polyurethane foams that were approximately twice as efficient per inch of thickness as any other insulation. For comparison purposes, water blown foams typically had a K-factor of 0.24 BTU-in/hr-°F-ft2 (34.5 mW/m-K) while those made from CFC-11 were 0.11 BTU-in/hr-°F-ft2 (15.8 mW/m-K). This significant energy savings bolstered CFC-11 as the supreme blowing agent for over three decades.
However, it was later found that CFCs had a negative environmental impact. It was mostly attributed to CFCs breaking down to chlorine radicals that attack and destroy several ozone molecules. As government regulations forced a phase out of CFCs, the next generation of blowing agents introduced to the industry were HCFCs (hydrochlorofluorocarbons).
Second Generation: HCFCs (Hydrochlorofluorocarbons)
Due to growing environmental concerns with CFCs, the fluorochemical industry offered two new blowing agent replacements: HCFC-141b and HCFC-123. As scientists moved to qualify and make new products, it was found that R-123 presented toxicity concerns and was thus ruled out. The next best alternative
was then R-141b (1,1-dichloro- 1-fluoroethane). R-141b has a gas lambda of 10 mW/m-K and presented unique formulation challenges attributed to a stronger solvency than R-11, which necessitated co-blowing 141b with water; both factors resulted in poorer insulation values. The industry subsequently optimized formulas around R141b, which were used in the United States for over a decade before another legislative directive went into effect. While HCFCs have been successfully phased out in non-article 5 countries, Article 5 countries continue to use them, albeit with imposed limits. Specific timelines and imposed restrictions vary depending on the country.
Third Generation: HFCs (Hydrofluorocarbons)
The next transition to HFCs was catalyzed due to increasing concerns on Ozone Depletion. Once again, the fluorochemical industry needed to innovate yet another alternative. The result from those research efforts were HFCs 134a, 245fa, and 365mfc. HFC-134a (1,1,1,2-tetrafluoroethane) and 245fa (1,1,1,3,3-pentafluoropropane) are gases at room temperature (25°C/77°F). HFC-365 mfc (1,1,1,3,3-pentafluorobutane), on the other hand, is a liquid at room temperature. A patent situation restricted the United States to use ONLY 245fa, and the European Union to use ONLY 365mfc. However, due to a force majeure on 365mfc caused by production issues, Europe was granted an exception that allowed their use of 245fa. The US was eventually granted the use of 365mfc until 2014. These alternatives presented their own unique challenges to formulators, particularly in thermal efficiency. These HFCs had inherently higher gas lambda values: HFC- 245fa at 12.7mW/m-K, HFC-365mfc 10.6 mW/m-K, and HFC-134a is 13.7mW/m-K.
As one can infer, it is evident that the historic trend has been that each successive blowing agent generation has shown poorer insulation capabilities. Table 1 summarizes the thermal conductivity of past and present fluorinated blowing agents used in the industry:
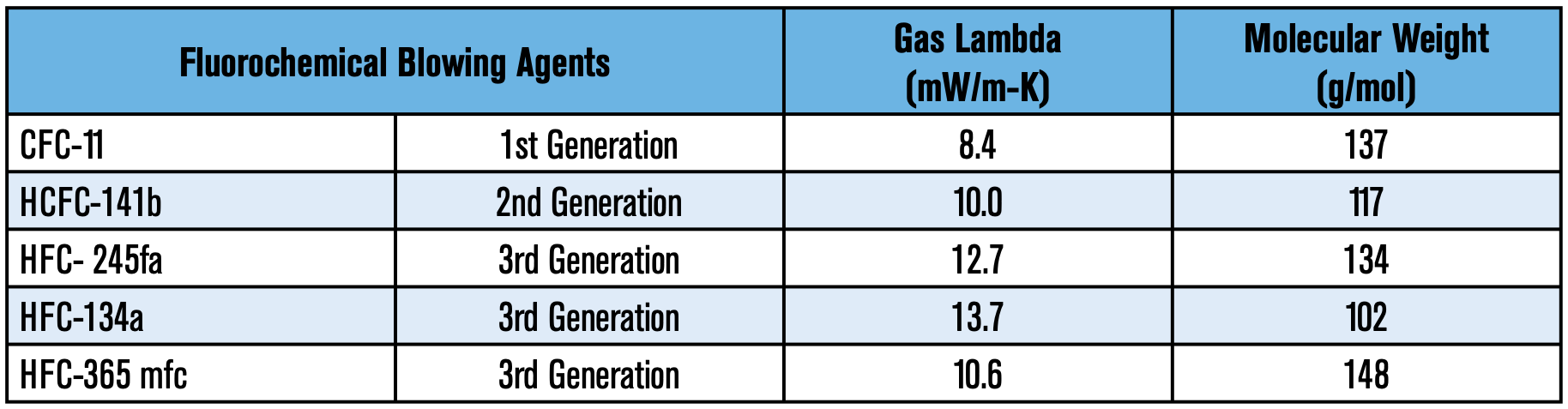
Fourth Generation Blowing Agents: The Challenges Ahead
While the appliance industry has successfully adapted to all blowing agent transitions, it has not been without compromise in efficiency and economics, not to mention the countless research efforts expended on optimizing formulations. In the world of polyurethanes, a new blowing technology is seldom a “drop-in” solution. The formulator must account for differences in boiling points, solubility, flammability, and stability, to name a few.
On top of that, the end-product must comply with ever-stringent energy efficiency demands. Fortunately, the polyurethanes industry has made vast improvements in polyols, surfactants, catalysts, and other additives that are part of the formulator’s toolbox to achieve the end goal. The top blowing agent candidates for the upcoming transition are presented in Table 2.
Before delving into each specific candidate, it is important to note that there is no such thing as a perfect blowing agent. Even across generations, each molecule has its merits and its shortcomings. The key to each successful transitory implementation has been through continuous optimization.
Optimization is crucial because thermal efficiency and overall performance is highly dependent on the formulation. It is not uncommon to see incompatibilities when directly swapping out blowing agents; what may have worked for one blowing agent may not work for another. Raw material components such as surfactants, as mundane as it may sound, have a tremendous impact on the foam’s performance—more on that later. One last thing to keep in mind is that a foam’s target density is calculated on a molar basis of the blowing agent. In other words, the higher the molecular weight, the more blowing agent is needed to achieve the same density.
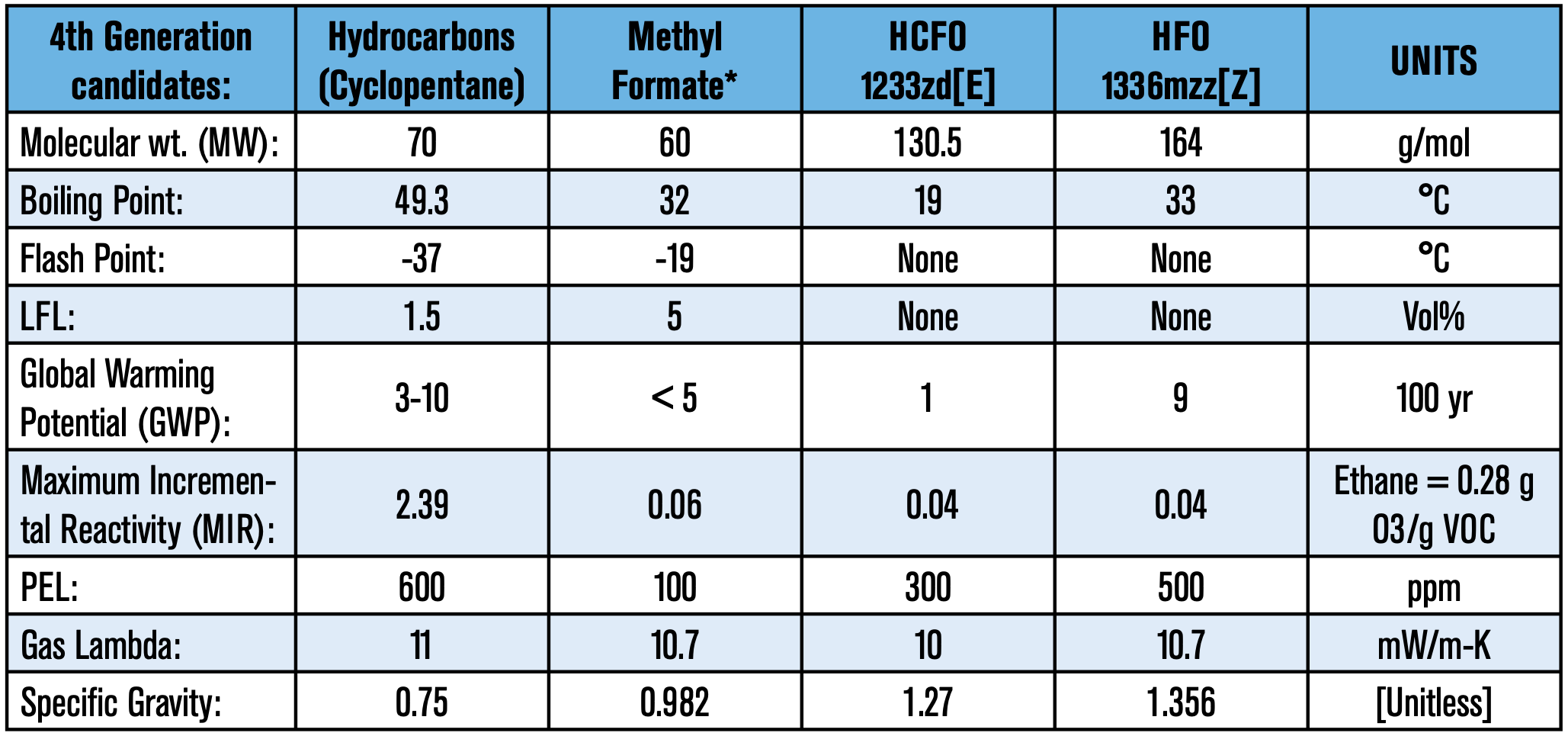
HCFO (HydroChloroFluoroOlefin), 1233zd(E):
HCFO 1233zd is a new blowing agent technology. One of the great advantages of this molecule is its low thermal conductivity; which appears to buck the historic trend of poorer lambdas with each succession. Below is a list of advantages and some potential disadvantages.
ADVANTAGES:
- Non-flammable
- Low GWP
- No smog issues with atmospheric life of 26 days
- Good thermal properties exhibited
- Moderate solubility so can use with a broad range of plastics
- Two manufacturers
POTENTIAL DISADVANTAGES:
- Marginal liquid at room temperature (boiling point: 19°C/66°F)
- Higher molecular weight (130.5 g/mol)
- Moderate economics, contains 3 fluorine atoms
- Unsaturation brought by C=C bond may present a shelf stability issue
- Specialty catalysts must be used, as conventional catalysts (e.g. PMDETA) have been reported to be highly unstable with this blowing agent
Ecomate (based on Methyl Formate):
Ecomate began to be used commercially in the early 2000s. It is a good choice for the formulator because of its low molecular weight and favorable economics. There are some drawbacks, however, such as its stronger solvency. It requires a different formulation approach, as dimensional stability and compressive strength can be an issue if not properly formulated. An analogous occurrence happened when formulators first used HCFC- 141b; once again the key to the success of both molecules has been optimization. As with hydrocarbon technology, methyl formate has been proven in the field and is currently sold in diverse market applications; especially applications which permit no compromise in thermal efficiency and energy consuming properties. Below is a list of advantages and disadvantages:
ADVANTAGES:
- Liquid at room temperature (boiling point: 32°C/89°F)
- Lowest MW; fewer quantities are needed to produce equivalent densities
- Low toxicity profile and excellent environmental profile: no ODP, low GWP, VOC exempt
- Cost efficient choice for SMEs
- No flammability load transferred to foams
- Excellent thermal properties; shows thermal synergy with HCFOs and HFOs
- Not a synthetic molecule
DISADVANTAGES:
- Is flammable in NEAT form, rated combustible when blended in fully formulated polyol component
- Is a strong solvent; formulation approach requires increased crosslink density
Case Study: The Importance of Formula Optimization
It was mentioned earlier that raw materials such as surfactants play a key role in a foam’s performance. To help illustrate the importance of raw material selection, a bench test on a basic formulation was executed in a laboratory hand-mix scale. The experimental approach was to find a suitable polyol combination that would yield good flow-ability and dimensionally stable foams. The next step was then to evaluate different surfactants to determine which would yield optimum thermal properties. The formulation used for this study is listed in Table 3:
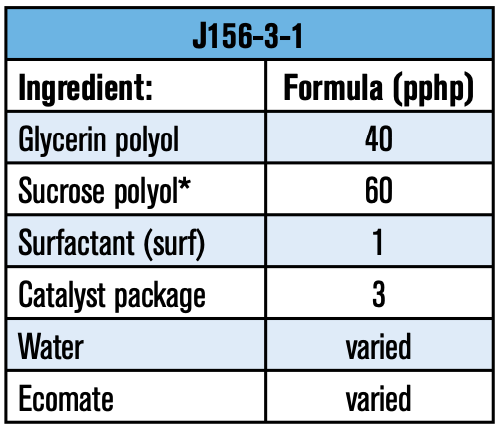
Polyol evaluations: One of the major components in a polyurethane foam is the polyol. Empirical evidence has shown that polyol selection influences important physical properties such as flow, lambda value (K-factor), dimensional stability, and/or shrinkage to name a few. In this study, different polyol packages (pkg) with a few sucrose polyols were examined with neat ecomate to assure that the foams had sufficient crosslinks to resist shrinkage (refer to Table 4).

During the screening process, Sucrose3 displayed a high level of shrinkage despite its 4.5 functionality. It is likely that the ratio of sucrose to glycerin of the latter was not adequate for this type of formulation.
Surfactant evaluations: The choice of surfactant is critical aspect in the optimization of a polyurethane foam formulation. Surfactant has a significant influence on cell size, flow characteristics, and most importantly, thermal insulation performance. A change in solubility caused by a change of the blowing agent will affect the surface tension of the foam cell windows – and dramatically affect the thermal conductivity of the foam. Equally important to the type of surfactant, is the amount of surfactant used… to much or too little can result in poorer lambda values.
To help demonstrate how influential a surfactant can be, Figure 1 shows a conventional formula—from a separate study—which was run with two different surfactants at the same loading level.
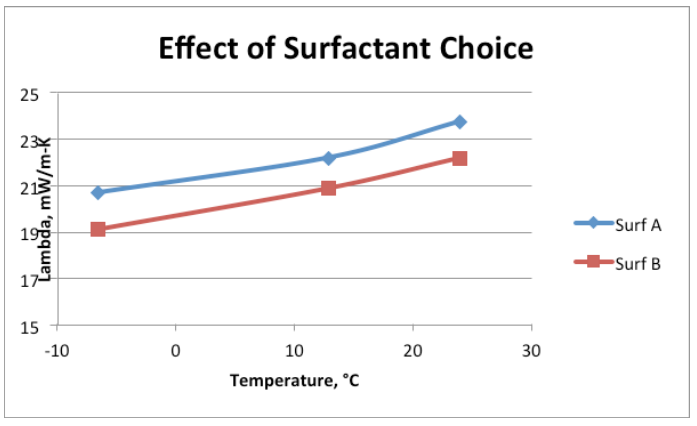
Based on this knowledge, we returned to the lab to evaluate polyols and surfactant combinations to optimize flow and minimize thermal conductivity in a low-density foam. We chose 3 surfactants to be screened against the three polyols shown in Table 4. It is important to state that lambda values are often inferior in hand-mix foams than from those dispensed through equipment; this case is not an exception. Further, we slowed down the reactivity of the foam system to allow for enough time to make foam samples for thermal conductivity and to evaluate flow properties on a small scale. As a side note, empirical tests show that slowing down reactivity can be deleterious to obtaining a lower lambda value.
Table 5 and Figure 2 illustrate how the polyol and surfactant choices both have strong influence on thermal properties. Note that the lowest lambda value represents the best insulation performance. As testing progressed, surf3 was ruled out, as it gave consistent high lambda values and cut down the overall number of trial runs. Results of one of the leading polyol package combinations (polyol pkg 6 with Surf1) is included for comparison purposes.
![Next-Generation Appliance Foams: The Challenges Ahead 7 Table 5: Effects of Polyol and Surfactant choice on Thermal Properties [Hand-mix Data]](https://fsi.co/wp-content/uploads/2024/01/Thermal-conductivity-results-of-different-polyol-packages.png)
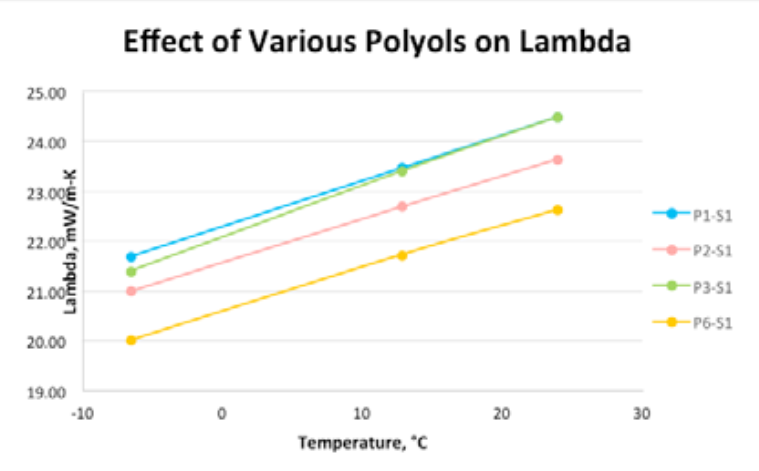
Appliance Foam
Synergy of Blowing Agent Blends
It is worth mentioning that formulators are also evaluating blowing agent blends. Blends have been used in the past, particularly to enhance properties, modify reaction rates, mitigate flammability, and/or to improve economics.
Field and lab trials completed by FSI over the past 10 years have demonstrated that methyl formate (ecomate) has thermal efficiency synergies when blended with HFO, HCFO and even hydrocarbons. Good thermal insulation results have been obtained with hydrocarbon/methyl formate blends that are equal or better than HFO or HCFO alone. And while this is indeed a flammable blend, appliance manufacturer production lines are already geared to handle this, since hydrocarbons are used today.
Similar thermal synergies of HFO/ HCFO blended with methyl formate have been reported by other parties as well. In one of those studies, a reported foam lambda value of 19.7 mW/mK at 24°C was reported with 1336mzz/methyl formate—a value that could improve with further optimization. With the new transition upon us, the industry will continue to investigate blends with these 4th generation blowing agent alternatives.
The outlook for rigid insulating foams is promising. The formulator counts on a diverse toolbox of raw material options to design and optimize the next generation formulas. The choice of surfactant, polyols, blowing agents, and combinations thereof will be essential to a successful transition. There is much work to be done, as optimization necessitates extensive research and experimentation. With a phase out date already scheduled, the time to start is today!
For further information, we recommend reading:



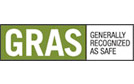

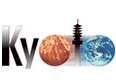
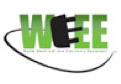
Unmatched Regulatory Compliance
Because it is environmentally benign, Ecomate complies with all current — and most likely all future — regulations pertaining to foam blowing agents. As additional HFCs and other harmful materials are phased out, Ecomate will continue to be the reliable and affordable solution for foam blowing applications. Current regulatory compliance includes:
Technical Support
We staff an industry-leading R&D team and a technical sales and support staff dedicated to building trusted industry relationships.
Together, these experts provide critical product and equipment guidance that allows appliance manufacturers to engineer more efficient, more sustainable household products.
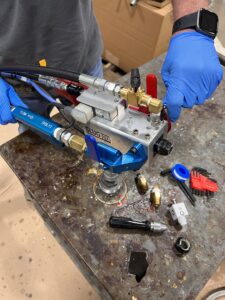